The Discovery and Properties of Arbutin
Originally isolated from plants like bearberries, Arbutin, scientifically referred to as 4-Hydroxyphenyl-β-D-pyranoglucoside, is a type of glycoside compound. Its presence was first detected in 1993 within the leaves of the Bergeniacrassifolia plant. Subsequently, it has been identified in various plants including blueberries, cranberries, bearberries, and most recently in the bark and leaves of pear trees.
Arbutin demonstrates a variety of pharmacological activities such as diuretic and antibacterial effects and has shown a cough-suppressant effect equivalent to codeine. Research conducted by Maeda et al. has shown that Arbutin is able to inhibit tyrosinase in melanocytes within a non-toxic concentration range, blocking the synthesis of dopa and dopaquinone, and thus suppressing melanin production. This mechanism gives Arbutin its skin-whitening effects.
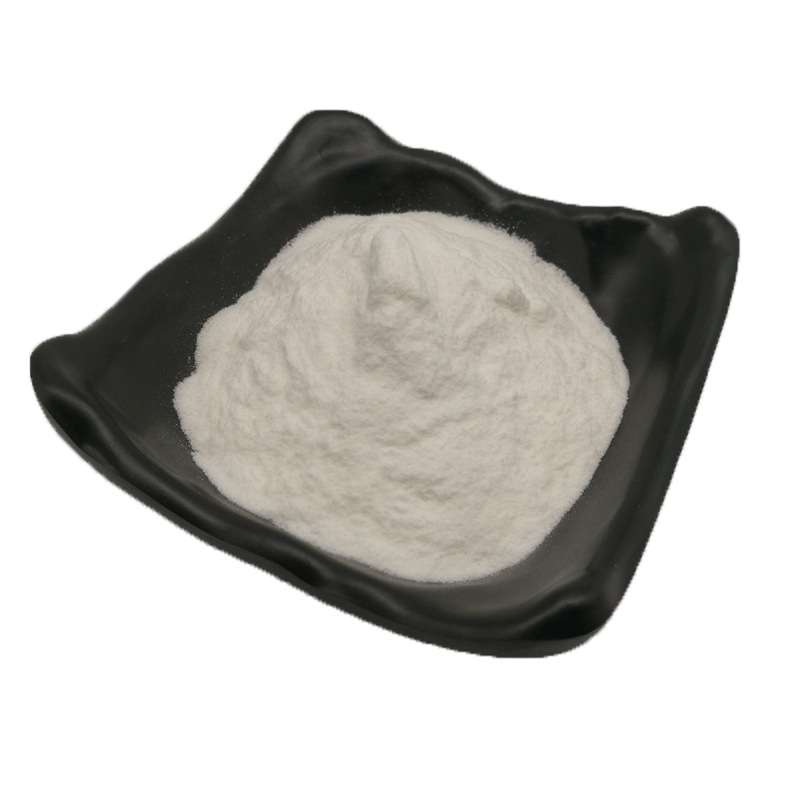
The Whitening Effect and Applications of Arbutin
Further studies by Kazuhisa and his team discovered that α-Arbutin (4-Hydroxyphenyl-α-D-pyranoglucoside) possesses a whitening effect that is more than ten times that of β-Arbutin, without inhibiting human cell growth or causing side effects. Furthermore, Arbutin can be mixed with other depigmentation agents like aloin to treat pigmented disorders synergistically, improving treatment outcomes. Due to its safety and significant whitening effects, Arbutin has been widely used and researched since the early 1990s when it was first employed in cosmetics as a whitening additive in Japan. There is a large demand for Arbutin both domestically and internationally.
Sources and Production of Arbutin
Arbutin was initially extracted from natural bearberries. Its sources now include plant extraction, biotransformation, organic synthesis, and enzyme synthesis. While natural extraction methods face challenges like low Arbutin content in plant material, low yield, complex extraction processes, and low product purity, these methods are currently unsuitable for large-scale industrial production. Synthetic products, due to their high purity, light color, high activity, and low production cost, dominate the market.
Organic Synthesis of Arbutin
Typically, the general steps of Arbutin’s chemical synthesis involve glucosylation of glucose and hydroquinone after appropriate protection, followed by deprotection. Early foreign literature records that the chemical synthesis of Arbutin required a β-D-pentadactyl glucose intermediate step. Classical Koenigs–Knorr reactions were employed, with bromo-glycoside as the donor, promoting condensation with mono-benzyl hydroquinone under the influence of silver salt, followed by deacetylation or debenzoylation to yield Arbutin. This method is no longer used in labs due to the expensive silver salt reagent and numerous reaction steps. Currently, most industrial synthesis methods for Arbutin adopt the Helferich method, using an acyl group as the donor.
Various improved methods of Arbutin synthesis have been reported in research, addressing challenges like unstable bromoacetate glucose intermediates, stringent reaction conditions, and complicated separation methods, which make these approaches unsuitable for large-scale production. Despite these advances, more research is needed to develop a more efficient, due to too long content.
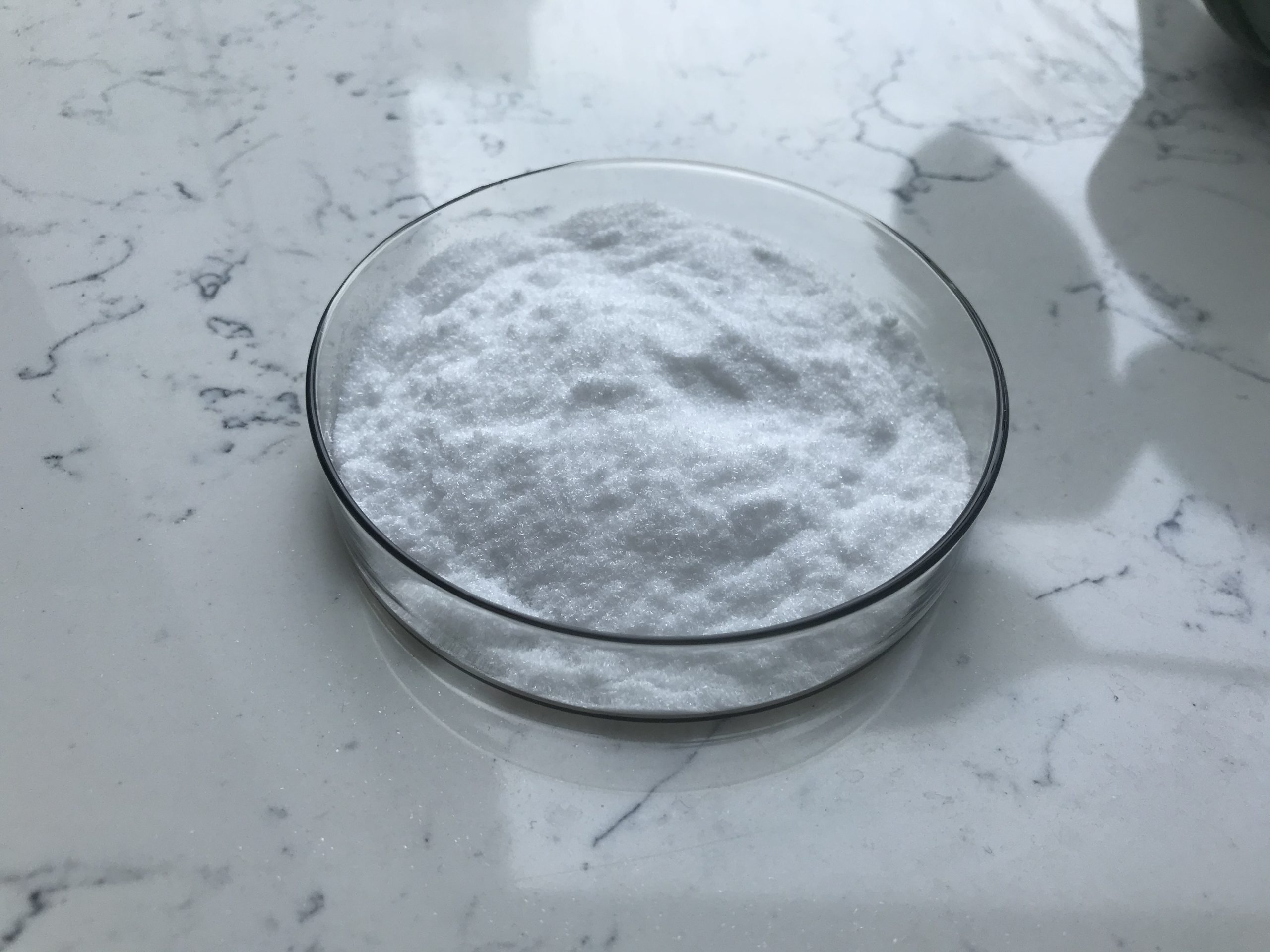
Arbutin: Enzymatic Synthesis and Biological Conversion
Enzyme Synthesis Method
Glycosyltransferase and glycosidase enzymes can be used in the enzymatic synthesis of Arbutin by catalyzing glycosyl transfer and reverse hydrolysis reactions. Though this method has a simple process and low production costs, it has a lower yield due to weak reactions. Consequently, the research on enzymatic synthesis mainly focuses on enzyme-mediated glycosyl transfer reactions.
Kurosu and colleagues achieved the synthesis of α-Arbutin from maltose and hydroquinone, catalyzed by freeze-dried cells of Pseudomonas campestris wu-9701. This selective glycosylation reaction led to no other byproducts and had a high hydroquinone conversion rate of 93%. Further research attempted to synthesize α-Arbutin from maltose and hydroquinone through a selective glycosyl transfer reaction in an aqueous phase of a sodium citrate-phosphate buffer system, using baker’s yeast as a biocatalyst. While the yield was not high, these enzymes can serve as appropriate biocatalysts for the synthesis of α-Arbutin and can be used more broadly in the α-glycosylation of multihydroxy phenolic compounds.
In research by Kazuhisa and colleagues from Japan, α-Arbutin and starch were used as glycosyl acceptors and donors. Through cyclodextrin glucanotransferase catalyzed stereoselective glycosyl transfer reaction, they synthesized α-glycoside of α-Arbutin. These new α-Arbutin derivatives not only have skin whitening effects, but due to the addition of the large glycosyl molecule, they also control the α-Arbutin’s long-lasting penetration into the skin. In addition, Arbutin derivatives with various glycosyl polymers can be synthesized in one pot by using glycosylation enzymes in a reaction of glucose residue of Arbutin and horseradish peroxidase-induced copolymerization of Arbutin with glycosyl Arbutin.
Enzymatic synthesis methods offer mild reaction conditions and clean, non-toxic products, making them promising for applications in the food and cosmetics industries. Due to their high efficiency and specific glycosylation reactions, they are especially suitable for α-Arbutin synthesis, compensating for the limitations of chemical synthesis methods. However, more research is needed to find and obtain suitable enzymes on a large scale and to improve the high-efficiency expression of key enzymes through genetic engineering. Simultaneously, research into synthesizing various Arbutin derivatives and finding simpler methods for Arbutin preparation and application is also a future research direction.
Biological Conversion Method
The biological conversion method involves the efficient transformation of raw materials into target products using the enzyme system in organisms. Currently, plant cells’ potent glycosylation capability is used to transform exogenous hydroquinone into Arbutin through cell and tissue culture methods.
Inomata and colleagues introduced hydroquinone into Periwinkle plant cell suspension, yielding high concentrations of Arbutin. Their experiments showed that the stage of cell culture growth and the method of adding hydroquinone had a significant impact on the conversion rate. Research by Yokoyama and others found that changing fresh culture medium and adding antioxidants could greatly increase the yield of Arbutin.
Recently, Liu Chunqiao and colleagues used a fermentation process, with Pantoea ananatis BT-112, to biologically synthesize Arbutin. They found that sucrose served as the best glycosyl donor, with the production rate of α-Arbutin relative to hydroquinone reaching up to 91.2%. After separation and purification using a macroporous adsorption resin, they obtained a product with a purity of over 99%. This process, which uses Pantoea ananatis BT-112 with a broader range of glucose donors than other strains, reduces production costs and lays a foundation for industrial production.
In biological conversion methods for synthesizing Arbutin, the selection of efficient plant tissue culture fluids and the determination of appropriate conversion conditions are critical. Different types of plant tissue cultures have significantly different conversion rates for hydroquinone. Future research needs to focus on understanding the growth mechanism of plant cells, identifying key influencing factors in the synthesis process, shortening the production cycle, and improving the ability and scale of biological conversion.
Quality Control
While artificial synthesis methods have overcome many shortcomings of natural extraction techniques for Arbutin, they often involve hydroquinone, a harmful substance to the human body. Thus, the quantitative and qualitative detection of the synthesized product and control of the quality of Arbutin is essential. For industrial production and application, the purity of Arbutin should be ≥99% as per the Ⅱ (Q/XXHG02—2002) standard. The phenol content should be <2×10-5, and the heavy metal content should be <1×10-5. As for the Ⅲ (Q/XXHG03—2002) standard, apart from the heavy metal content, which should be <1×10-5, the purity requirement is higher, set at ≥99.5%, and the phenol content should be <1×10-5.
Spectroscopic Methods
Arbutin solutions stored in the air are sensitive to storage time when measured with fluorescence and UV absorption spectroscopy, making them unsuitable for long-term concentration monitoring. Fei Li et al. [25] used Fourier Transform Infrared Spectroscopy and UV absorption and fluorescence spectroscopy under nitrogen protection to monitor Arbutin concentrations, demonstrating good measurement accuracy and stability. When measuring three groups of standard concentration samples, the relative measurement errors of the three methods were less than 7%, 2.6%, and 2%, respectively.
High-Performance Liquid Chromatography
High-Performance Liquid Chromatography (HPLC) has been widely reported for measuring the Arbutin content in plant materials and cosmetics [26,27]. Ting Zhang et al. [28] established an HPLC method for determining the content of Arbutin in the gel. They used a Polaris C18 column, methanol-water mixed solvent as the mobile phase (volume ratio of methanol/water 20∶80), and phenol as the internal standard. In the concentration range of 5~160 μg/mL, the peak area ratio of Arbutin to the internal standard showed a linear relationship with its concentration. The average recovery rate was 99.7%, and the RSD was 0.60%. Reports on determining the types and distribution of impurities in synthesized products to guide the synthesis and purification of Arbutin are scarce.
Yuna Wei et al. [29] established a method for determining the content of phenol in synthesized Arbutin using HPLC. They also determined the molecular formula of an unknown impurity in Arbutin through LC-MS technology and preliminarily deduced its possible structural formula. This method allows for the simultaneous determination of the content of Arbutin, phenol, and other impurities. By measuring the impurities in Arbutin products from ten different batches, they established a chromatographic fingerprint of Arbutin through data analysis and statistics. By using a similarity calculation method (Euclidean distance), they were able to assess the quality of the products and guide the synthesis, purification, and quality control processes.
Conclusion
Among the three synthesis methods of Arbutin, enzymatic and biological transformation methods are not easily adaptable for industrial production. Due to problems such as low substrate concentration and long reaction time, enzyme-catalyzed synthesis cannot be realized on a large scale. Although tissue culture methods of biological transformation have high efficiency, their scale is still small, the cultivation time is long, and the available enzyme system is limited. Large-scale production still has many problems to be solved. However, chemical synthesis can overcome these deficiencies, with advantages such as short synthesis time and high product yield, making it suitable for large-scale industrial production. But due to the potential for harmful phenolic substances to remain in the synthesized product, improving the selectivity of the reaction and advancing the separation and purification technology are key areas of future research.
There are few reports on the synthesis of Arbutin in China, and there is a certain gap compared with foreign countries in terms of synthesis and production. However, with the continuous improvement of people’s living standards and the increasing awareness of health and aesthetics, the application field of Arbutin will gradually expand, and the social demand for Arbutin will continue to rise. This will strongly promote in-depth research on the synthesis methods of Arbutin and its derivatives in China.
References
[1] Steglich W, Fugmann B, Lang S. New York: Georg Thieme, 1997, 53 [2] Budavari S O, Neil M J, Smith A. Eleventh, Rahway, Merck Co.In, 1989 [3] Cai T, Nakamura K, Ma L. Agric. Food Chem. 2005, 53: 3882~3887 [4] Frohne. Planta Med. 1969, 18(1): 1~25 [5] Meadd K, Fukuda M. J Pharmacol Exp Ther, 1996, 276(2): 765~769 [6] Kazuhisa S, Takahisa N, Kojin N. Chem. Pharm. Bull, 2003, 51(7): 798~801 [7] Kazuhisa S, Takahisa N, Koji N. Biol. Pharm. Bull, 2004, 27(4): 510~514 [8] Yang Z, Wang Z, Tu J. Chinese Journal of Aesthetic and Plastic Surgery, 2004, 20(5): 369~371 [9] Robertson A, Waters R B. J. Chem. Soc., 1930.5: 2729~2733 [10] Li W, Liu H, Zhang Y. Journal of Zhengzhou University of Technology, 1999, 20(2): 42~44 [11] Zhou F, Lu D, Wei P. Spices, Fragrances, Cosmetics, 2005.(4): 11~12 [12] Yao B, Chen W, Wu Q. Chinese Journal of Modern Applied Pharmacy, 2005, 22(5): 389~390 [13] Huang S L, Zhu Y L, Pan Y J. J. Zhejiang Univ, 2004, 5(12): 1 509~1511 [14] Wang Z X, Shi X X, Chen G R. Carbohydrate Research, 2006, 341: 1945~1 947 [15] Liu F, Jiang T, Ren S. Daily Chemical Industry, 2004, 34(4): 242~244 [16] Kurosu J, Sato T, Yoshida K, Tsugane T, Shimura S, Kirimura K, Kino K, Usami S. Journal of Bioscience and Bioengineering, 2002, 93(3): 328~330 [17] Prodanovic R M, Milosavic N B, Sladic D, Velickovic T C, Vujcic Z. Biotechnology Letters, 2005, 27: 551~554 [18] Sugtmoto K, Nomura K, Nishimura T, Kiso T, Sugloto K, Kuriki T. Journal of Bioscience and Bioengineering, 2005, 99(3): 272~276 [19] Nakano H, Shizuma M, Murakami H, Kiryu T, Kiso T. Journal of Molecular Catalysis B: Enzymatic, 2005, 33: 1~8 [20] Yokoyamo M, Inomam S. JP 92 131 091, 01. May, 1992 [21] Noguchi N, Horiuchi T, Hattori R, Sato H. JP 5 176 785, 20. Jul. 1993 [22] Zhao M, Ding J, Liu J. Chinese Journal of Traditional Chinese Medicine, 2001, 26(12): 819~822 [23] Liu C, Zhang S, Zhang P. Daily Chemical Industry, 2005, 35(6): 364~367 [24] Jahodar L, Duskova J, Polasek M. Czech Rep, Pharmazie, 1999, 54(3): 234~235 [25] Li F, Gong Y, Zhang X. Analytical Chemistry, 1999, 27(1): 119 [26] Masse M O, Duvallet V, Borremans M. International Journal of Cosmetic Science, 2001, 23(4): 219~232 [27] Lin C H, Wu H L, Huang Y L. Journal of Chromatography, B: Analytical technologies in the biomedical and life Sciences, 2005, 829(12): 149152 [28] Zhang T, Li X, Zhou B. Journal of Guangdong College of Pharmacy, 2004, 20(6): 635~636 [29] Wei Y, Li D, Lian H. Chemical Industry Times, 2005, 19(7): 11~14
3-O-Ethyl Ascorbic Acid AHK-Cu Alpha Arbutin Alpha Arbutin Powder azelaic acid Brazzein Chitosan Oligosaccharide Coenzyme Q10 collagen copper peptide powder Cycloastragenol D-panthenol Dutasteride Powder finasteride food additives Fullerene C60 Fullerene C60 Powder Hyaluronic Acid Powder Magnesium Ascorbyl Phosphate Melatonin NMN Powder Phycocyanin RU58841 RU58841 powder Sucralose Powder Thaumatin thaumatin powder TUDCA vitamin K2 WS-23